A path from bench to bedside in sudden death in epilepsy (SUDEP) – an opinion from Mark Stewart
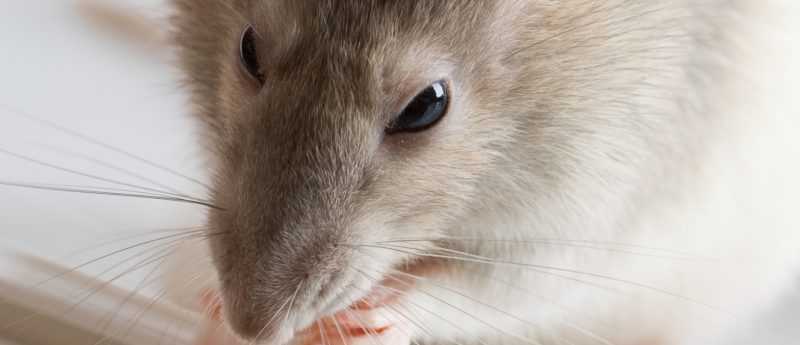
Background Sudden death in epilepsy (SUDEP) has received considerable attention in the last decade, resulting in refinements in the definition (“… a non-traumatic, non-drowning death that occurs in benign circumstances in an individual with epilepsy …” See e.g. [1,2]), more detailed calculations of incidence (from approximately one to nine deaths per 1000 patient years) [2–4], and the identification of key cardiopulmonary events that contribute to an overall pattern ending with death [3]. A wide range of animal models has been used to explore cardiac and/or respiratory derangements due to or associated with seizure activity that may contribute to an individual’s...